Impact of diet and gut microbiota changes in the development of hepatocellular carcinoma
Abstract
Hepatocellular carcinoma (HCC) is a primary liver cancer that occurs with a frequency of 85% in patients with liver cirrhosis. It is the sixth most common type of cancer globally. Asia is the continent with the highest incidence (72%), followed by Europe (8%) and Africa (5%). Men are four times more likely than women to develop this cancer, especially in the 70-80 age group. Risk factors include alcoholic liver disease, tobacco use, genetic predisposition, dysmetabolic comorbidities such as type 2 diabetes mellitus and obesity, hepatitis B virus and hepatitis C virus infections, and non-alcoholic fatty liver disease. Unhealthy dietary regimens and gut dysbiosis are additional risk factors that have been recently investigated. These two factors are closely related because the gut microbiota performs several biological functions, including nutrient metabolism, a process that promotes gut homeostasis, known as eubiosis. With regard to the correlation between diet, gut microbiota, and HCC development, there are several mechanisms that have not yet been fully elucidated. This narrative review aims to evaluate the impact of diet and gut microbiota changes in the development of HCC. Our analysis, performed on several clinical and pre-clinical studies, showed that a high-fat diet promotes gut dysbiosis and hepatic fat accumulation, leading to the progression from simple steatosis to HCC, while the Mediterranean diet, rich in fiber and monounsaturated fatty acids, had a protective role. For this reason, international employment of this dietary regimen for therapeutic purposes should be encouraged.
Keywords
INTRODUCTION
Hepatocellular carcinoma (HCC) is a primary liver cancer that occurs with a frequency of 85% in patients with liver cirrhosis[1,2]. Men are four times more likely than women to develop HCC, especially in the 70-80 age group[3]. Risk factors are alcoholic liver disease (ALD), tobacco use, genetic predisposition, type 2 diabetes mellitus (T2DM), obesity, hepatitis B virus (HBV) and hepatitis C virus (HCV) infections, and non-alcoholic fatty liver disease (NAFLD)[4,5]. In the case of HCV infection, mutations in the viral genome that prevent the achievement of a sustained virologic response after antiviral therapy are increasingly common[6-8]. This event is closely related to the circulation of specific viral genotypes[9]. With regard to HBV, its prevention through vaccination has been successful in globally reducing the incidence of HCC[10]. NAFLD represents a possible first stage of liver damage with a specific natural history ranging from accumulation of fat in the liver to necroinflammation, fibrosis, cirrhosis, and HCC[11]. Recently investigated additional risk factors are an unhealthy dietary regimen and gut dysbiosis: these two factors are closely related because the gut microbiota performs several biological functions, including nutrient metabolism, a process that promotes gut homeostasis, known as eubiosis[12]. For instance, the gut microbiota was recently involved in the treatment of HCC[13-15]. Changes in gut bacteria abundance in correlation with the progression from NAFLD to HCC have been investigated in recent studies. In particular, an increase in Ruminococcus and Escherichia and a decrease in Lactobacillus and Bifidobacterium have been seen in patients with advanced fibrosis[16]. In addition, alcohol-associated dysbiosis is linked to a reduced biosynthesis of long-chain fatty acids by the Lactobacillus genus[17]. At the same time, infective liver cirrhosis showed an increased abundance of Prevotella, Streptococcus, Staphylococcaceae, and Enterococcus, as well as decreased Ruminococcus and Clostridium[18]. Certain dietary regimens have been proven to have a positive effect on liver diseases such as NAFLD; in particular, the Mediterranean diet is rich in monounsaturated fatty acids, which reduce risk factors for metabolic syndrome related to NAFLD, such as waist circumference, high-density lipoproteins, and triglycerides, and has been shown to grant a protective effect against cardiovascular events[19-22]. Risk factors for HCC development are summarized in Figure 1.
Figure 1. Risk factors for HCC development. ALD: alcoholic liver disease; NAFLD: non-alcoholic fatty liver disease; HCC: hepatocellular carcinoma.
As for the correlation between diet, gut microbiota, and HCC development, there are several mechanisms that have not yet been fully elucidated. This narrative review aims to evaluate the impact of diet and gut microbiota changes in the development of HCC.
DIET AND HCC DEVELOPMENT
Diet refers to the total amount of food individuals consume[23]. In this context, the gut is involved in the fermentation of carbohydrates as it is related to short-chain fatty acids (SCFAs) production, cleavage of proteins into amino acids, synthesis of vitamins, and metabolism of polyphenols[24]. Diet plays a dual role in HCC development: while a diet rich in polyphenols, fiber, and omega-3 grants a protective effect, a diet high in saturated fat, red meat, and fried food may predispose to the development of the disease[25].
Junk food and HCC
Unhealthy foods, high in calories and low in nutritional value, are referred to as “junk food”[26,27]. Current literature lacks clinical data about the impact of junk food on the development of HCC. However, a recent study was performed by Hymel et al. on non-alcoholic steatohepatitis (NASH)-HCC mice models fed with junk food[28]. The Authors reported that a diet high in trans fat, cholesterol, and fructose contributes to HCC development in both lean and obese mice. The design of these mice models of NASH-HCC may be of critical importance for new studies in humans in the near future. Overall, a dietary regimen with junk food promotes the development of metabolic comorbidities, which supports tumorigenesis. At the same time, adipocytes become hypertrophic due to lipid accumulation and activate a series of biochemical pathways, including the overproduction of oxygen-free radicals (ROS) and the synthesis of pro-inflammatory cytokines. In addition, pro-inflammatory cytokines activate ROS production by monocytes and macrophages, promoting systemic inflammation and creating an ideal tumor microenvironment[29].
Ketogenic diet and HCC
The ketogenic diet, characterized by a high fat and a low carb intake, prompts ketosis[30-34]. However, data on its impact on HCC are scarce. Healy et al. studied mice fed ketogenic, obesogenic, or control diets, revealing lower tumor burdens in the ketogenic group, regardless of obesity[35]. Elevated interleukin-6 levels correlated with tumor burden, while serum adiponectin inversely was related to sugar intake. Huang et al. further investigated ketone body exploitation by HCC, revealing OXCT1’s correlation with HCC staging[36]. Ketone body catabolism in HCC cells promoted ATP increase and inhibited AMP-activated protein kinase activation. Byrne et al. showed no significant HCC staging alterations in mice fed with ketogenic diets[37]. Despite delayed initiation, limited glucose availability effectively hindered cancer cell reliance on glycolysis. The clinical study performed by Motta et al. supported findings in mice models, showing tumor disappearance and clinical improvements after ketogenic diet monotherapy. Furthermore, blood ketone bodies rose, while glucose levels decreased, emphasizing the diet’s potential to limit tumor growth by reducing glucose availability[38]. These studies collectively shed light on the ketogenic diet’s potential in HCC management, suggesting further research is needed for comprehensive understanding and application.
Mediterranean diet and HCC
The Mediterranean diet was conceptualized by Ancel Keys in the 1960s as a diet that is low in saturated fat and rich in vegetable oils[39,40]. This diet grants several beneficial effects by reducing the risk of cardiometabolic comorbidities and liver diseases[41-44]. However, there are few clinical studies about its role in preventing the development of HCC. The first study was performed by Turati et al., who evaluated the possible protective effect of the Mediterranean diet in 518 patients with HCC and 772 controls[45]. The study showed that HCC patients were less adherent to the Mediterranean diet. Furthermore, strong adherence to this dietary regimen disfavored the development of HCC among patients with liver cirrhosis of infectious etiology. In the same year, Li et al. assessed the risk of developing HCC in a cohort of 494.942 enrolled patients[46]. Higher adherence scores were associated with a lower risk of HCC and chronic liver disease mortality. Overall, a strong commitment to the Mediterranean diet reduced the incidence of HCC. Likewise, Ma et al. evaluated the impact of different dietary scores in a large cohort of patients[47]. The results showed that adherence to the Mediterranean diet did not correlate with the development of HCC. Bogumil et al. performed an observational study with a more focused approach to ethnic groups[48]. The dietary regimen of 169.806 individuals belonging to different ethnic groups was analyzed with a clinical follow-up of 17 years. The results showed that a better adherence to the Mediterranean diet reduced the incidence of HCC. This can be explained by its composition, as it primarily comprises foods that are abundant in polyphenols, known for their antioxidant and anti-inflammatory properties. These substances decrease the concentration of ROS and suppress the proliferative pathways of phosphatidylinositol 3-kinase, mitogen-activated protein kinases (MAPK), and nuclear factor kappa B (NF-kB). Furthermore, vitamins C and E, along with flavonoids, prevent DNA damage. The high content of omega-3 reduces cell proliferation, the inflammatory process, and tumor angiogenesis. Moreover, a limited intake of fats hinders the development of hyperinsulinemia and consequently inhibits the growth of cancer cells[49].
Alcohol intake and HCC
The mechanisms underlying alcohol-related hepatocarcinogenesis are multifactorial. As such, chronic alcohol exposure leads to oxidative stress, DNA damage, and impaired DNA repair mechanisms, which promote the accumulation of genetic mutations and genomic instability, the two hallmarks of cancer development[5,50]. Additionally, alcohol metabolism generates acetaldehyde, a highly reactive and toxic compound that can directly damage DNA and proteins, further contributing to carcinogenesis. Moreover, alcohol-induced liver injury triggers compensatory regenerative responses, leading to the proliferation of hepatocytes and the activation of hepatic stellate cells, which promotes fibrogenesis and creates a pro-carcinogenic microenvironment. Chronic inflammation further fuels carcinogenesis by promoting cell proliferation, angiogenesis, and evasion of immune surveillance. Importantly, the risk of HCC development in individuals with ALD is influenced by various factors, including the duration and intensity of alcohol consumption, concomitant viral hepatitis, and genetic predisposition[51,52]. Furthermore, several factors contribute to the heightened risk of HCC in individuals with combined ALD and NAFLD, especially in individuals with dysmetabolic comorbidities[53]. The combined insult of alcohol-induced liver injury and metabolic dysregulation can synergistically promote hepatic inflammation, fibrosis, and carcinogenesis due to oxidative stress through the previously mentioned shared common pathways[54,55]. Table 1 shows the different pre-clinical and clinical studies on the development of HCC and different dietary regimens.
Summary of the different studies on the development of HCC and different dietary regimens
Authors | Study model | Dietary regimen | Outcome |
Hymel et al., 2022[28] | NASH-HCC mice | Junk food | Contribution of hepatocarcinogenesis in lean and obese mice |
Healy et al., 2014[35] | C57BL/6N mice | Ketogenic diet | Lower tumor burden compared with high-carbohydrate diets |
Huang et al., 2016[36] | HCC mice | Ketogenic diet | Early use of the ketogenic diet in mice models has an antitumor effect in the liver |
Byrne et al., 2018[37] | C57BL/6 mice | Ketogenic diet | A delayed dietary regimen did not lead to any benefit |
Motta et al., 2020[38] | Two patients with HCC | Ketogenic diet | Disappearance of the tumor, with significant clinical improvements after one year of therapy |
Turati et al., 2014[45] | 518 patients with HCC and 772 controls | Mediterranean diet | A strong adherence to the Mediterranean diet hindered the development of HCC among patients with liver cirrhosis of infectious etiology |
Li et al. 2014[46] | 494.942 enrolled patients; 509 incident cases of HCC | Mediterranean diet | Strong adherence to the Mediterranean diet reduced the incidence of HCC |
Ma et al., 2019[47] | 160 patients with incident HCC | Mediterranean diet | The adherence to the Mediterranean diet did not correlate with the development of HCC |
Bogumil et al., 2019[48] | 169.806 enrolled patients; 605 incident cases of HCC | Mediterranean diet | Strong adherence to the Mediterranean diet reduced the incidence of HCC, independently of ethnicity |
GUT DYSBIOSIS AND HCC
The gut microbiota plays an important role in keeping humans healthy through immunity and metabolic processes, but it is also related to several pathologies[56]. As such, it has been suggested that commensal bacteria may be intricately involved both in the pathogenesis and in the prevention of HCC. Gut homeostasis is linked to gut barrier status, with a possible disruption or dysfunction associated with local or systemic consequences[57]. Gut microbiota dysbiosis promotes the development of HCC by increasing the permeability of the gut barrier and triggering liver inflammation[58]. In particular, the leaky gut is defined by the intestinal barrier's increased permeability, which allows lipopolysaccharide (LPS) entry through the portal vein to the liver[59]. This results in the activation of hepatic natural killer T (NKT) cells and an increase in toll-like receptor (TLR) 2 expression by hepatic stellate cells and TLR4, triggering chronic inflammation that promotes liver fibrosis and tumorigenesis. Furthermore, the assessment of endotoxin levels and oxidant status is crucial in comprehending the pathophysiology of NAFLD and its complications. Serum soluble phagocytic NADPH oxidase 2 (sp-NOX2) and urinary 8-iso-prostaglandin F2 alpha
Gut microbiota changes in patients with HCC
Gut dysbiosis is found in HCC patients. One of the first studies was performed by Ponziani et al. in patients with liver cirrhosis and NAFLD[61]. A high level of fecal calprotectin was observed in HCC patients, which showed a higher abundance of Bifidobacterium and Akkermansia. Bacteria belonging to Ruminococcus and Bacteroides genera were more abundant in HCC patients compared to cirrhotic patients. A strong correlation between gut microbiota profile and systemic inflammation, a process that leads to tumorigenesis, was found among groups. Indeed, cirrhotic patients with NAFLD and HCC lacked protective bacteria, thus engendering a subsequent inflammatory process in the gut. In order to characterize the gut microbiota that triggers the transition from chronic liver disease to HCC, Effenberger et al. compared the bacterial profile of patients with HCC and liver cirrhosis to that of patients with NAFLD[62]. Fecal samples were collected from patients with liver cirrhosis and HCC; both showed a reduced abundance of different taxa, including SCFAs-producing genera such as Blautia and Agathobacter. Using 16S coupled ribosomal RNA gene and transcriptome sequencing, a direct association was identified between the abundance of the gut bacterial genus and the host transcriptome response within liver tissue. This evidence indicates that perturbations of the gut-liver resident microbiota are a critical determinant of patients with HCC. In these patients, the gut microbiota is characterized by potentially pathogenic bacteria and the development of liver disease is mediated by bacterial metabolites, such as the inhibition of
Gut microbiota changes in HCC patients after immunotherapy
Commensal bacteria may improve the efficacy of cancer therapy by inducing antitumor immune responses. Several studies showed that Bacteroides fragilis has a positive influence on immune checkpoint inhibitors (ICI) treatment through activation of CD4+ T cells and interferon (IFN)-γ producing dendritic cells[65,66]. Bifidobacterium, Bacteroides, Eubacterium, and Fusobacterium genera have also been shown to increase the efficacy of immunotherapy by raising IFNγ+ and CD8+ T cells’ responses[67]. Fecal samples from patients who responded to immunotherapy showed higher taxa richness than those from non-responders. Indeed, Akkermansia muciniphila and Ruminococcaceae were the most abundant in patients who responded to ICI[68]. According to the Authors, Akkermansia muciniphila degrades mucin to produce SCFAs with subsequent activation of G protein-coupled receptors and inhibition of histone deacetylases. The bacterial-induced signal transduction mechanism involves TLR-2, glucagon-like peptide 2, and natural killer cell group 2 member D ligand. This step leads to a decrease in pro-inflammatory cytokines by disfavoring the establishment of a suitable tumor microenvironment. It also promotes the integrity of the intestinal barrier, and for this reason, it is used as a next-generation probiotic. As for Ruminococcaceae, its synergistic action in the production of butyrate promotes intestinal balance. Furthermore, this evidence suggests the gut microbiota’s ability to promote an antitumor response by activating CD8+T cells, which are essential in controlling HCC growth. In this regard, early changes in gut microbiota could be analyzed to predict immunotherapy outcomes. In the study performed by Lee et al., supportive therapy with Bifidobacteria was used in patients with HCC undergoing perioperative hepatectomy[69]. According to linear discriminant analysis, HCC patients with progressive disease after ICI therapy showed a higher abundance of Prevotella, while Veillonella, Lachnoclostridium, and Streptococcaceae were the most abundant taxa in the responder's group. Bile acids showed significantly higher concentrations in patients who responded to ICI and were positively related to Lachnoclostridium relative abundance. The latter, acting in synergy with other intestinal microorganisms, promotes bile acid homeostasis. However, under imbalanced conditions of gut microflora and bile acid secretion, there is pathway blockage involving CYP7A1 and SHP, promoting fat synthesis. At the same time, an excess of secondary bile acids derived from the gut microbiota promotes the establishment of an inflammation process, with the activation of mTOR signaling in hepatocytes and subsequent tumorigenesis. Such evidence confirms the role of the gut microflora as an enhancer in immunotherapy[15].
INTERPLAY BETWEEN DIET, GUT MICROBIOTA AND HCC DEVELOPMENT
To date, most of the evidence on the role of different dietary regimens and gut microbiota in HCC development is based on functional studies in mice models[70]. More studies are needed to define the microbial and metabolite signatures of HCC in order to provide a potential therapy[71-73]. The metabolic role of the gut microbiota can influence several disease conditions, including liver cancer[74]. Yamada et al. assessed the promotion of HCC development using high-fat diets that induce steatohepatitis (STHD-01) in mice models[75]. Mice fed the STHD-01 diet developed HCC and, subsequently, HCC after 9 and 41 weeks. The impact of the gut microbiota was highlighted by antibiotic treatment, which not only dramatically reduced the accumulation of secondary bile acids but also significantly reduced liver disease and suppressed the tumor. The bacterial genera Bacteroides and Clostridium cluster XVIII were increased, while the Bifidobacterium, Prevotella, and Streptococcus genera decreased. The cause triggering the development of HCC is related to the products of the gut microbiota after following the STHD-1 high-fat diet, such as secondary bile acids: specifically, an increase in the transcription of cytochrome P450 cholesterol 7 alpha-hydroxylase, which is involved in the synthesis of bile acids in the liver. 7α-hydroxycholesterol is converted to 7α-hydroxy-4-cholesten-3-one, which is the precursor for the synthesis of cholic acid and chenodeoxycholic acid. In line with the accumulation of cholesterol in the liver, the concentration of total bile acids was significantly higher in the plasma and feces of mice fed with the STHD-01 diet
Figure 2. Gut microbiota changes and progression to HCC in mice models fed with STHD-01(A) and rich in cholesterol diets (B). STHD: high-fat diets that induce steatohepatitis; NASH: non-alcoholic steatohepatitis; HCC: hepatocellular carcinoma; NAFLD: non-alcoholic fatty liver disease.
Recent evidence shows that an excessive presence of cholesterol in the alimentary regimen is the main factor responsible for HCC development. In fact, it reduces the abundance of Bifidobacterium and Bacteroides genera and promotes gut dysbiosis; at the same time, triglycerides and very low-density lipoproteins are produced. The latter transport triglycerides from the liver to tissues, but if they are not correctly formed, triglycerides accumulate in hepatocytes, inducing NAFLD, which in turn can advance into HCC
CONCLUSIONS AND FUTURE PERSPECTIVES
Diet and gut microbiota are the main actors in the development of HCC, from as early as the onset of liver steatosis. However, functional studies are necessary to highlight the metabolic pathways that lead to this evidence. A healthy diet such as the Mediterranean diet can prevent the onset of liver cancer. It is therefore necessary to use this dietary regimen for therapeutic purposes and to promote its adherence internationally. Concurrently, probiotics, prebiotics, and symbiotics should be used for preventive purposes to restore gut eubiosis, while another approach could be the use of cholesterol inhibitors to prevent HCC[84]. Finally, all microbiology laboratories should implement the use of gut microbiota sequencing to evaluate the changes in bacterial composition, mainly in critical area patients[85-87]. Shotgun metagenome sequencing, especially when combined with deep learning tools, quantifies the abundance of gut bacteria with high resolution[88,89]. The application of these data, together with clinical and laboratory parameters, is crucial in diagnosis and targeted therapies. Consequently, there is a significant shift toward the use of machine learning approaches in the genomics discipline[90-92]. At the same time, machine learning finds wide application as a predictive model of inflammatory bowel diseases in the gastroenterology field[93]. Diagnostic and therapeutic strategies focused on the gut microbiota are possible because of the continuous collaboration between gastroenterologists and microbiologists. In conclusion, we propose the following future perspectives in Table 2.
Take-home messages and future perspectives
Take-home messages | Future perspectives |
Diet and gut microbiota are involved in HCC development from as early as the onset of liver steatosis | Functional studies are needed to highlight the metabolic pathways between diet, gut microbiota, and HCC development |
Probiotics, prebiotics and symbiotics can be used for preventive purposes to restore gut eubiosis | Wide therapeutic use of the Mediterranean diet against HCC |
Application of gut microbiota analysis in each microbiology laboratory | Increased application of machine learning to genome sequencing techniques |
DECLARATIONS
Acknowledgments
We would like to thank Simone Scarlata for his critical review of the English language.
Authors’ contributions
Wrote, reviewed, and edited the manuscript: Scarlata GGM, Cicino C
Provided figures and tables: Scarlata GGM, Cicino C
Reviewed and approved the final manuscript as submitted: Spagnuolo R, Marascio N
Read and approved the final manuscript: Quirino A, Matera G, Dumitrașcu DL, Luzza F, Abenavoli L
Conceptualized and designed the review: Abenavoli L
Availability of data and materials
Not applicable.
Financial support and sponsorship
None.
Conflicts of interest
All authors declared that there are no conflicts of interest.
Ethical approval and consent to participate
Not applicable.
Consent for publication
Not applicable.
Copyright
© The Author(s) 2024.
REFERENCES
1. Llovet JM, Kelley RK, Villanueva A, et al. Hepatocellular carcinoma. Nat Rev Dis Primers 2021;7:6.
2. Singal AG, Lampertico P, Nahon P. Epidemiology and surveillance for hepatocellular carcinoma: new trends. J Hepatol 2020;72:250-61.
3. McGlynn KA, Petrick JL, El-Serag HB. Epidemiology of hepatocellular carcinoma. Hepatology 2021;73 Suppl 1:4-13.
4. Ge XY, Sun MC, Wang TY, et al. Analysis of risk factors of hepatocellular carcinoma and establishment of a clinical prognosis model. Front Oncol 2023;13:1067353.
5. Méndez-Sánchez N, Valencia-Rodriguez A, Vera-Barajas A, et al. The mechanism of dysbiosis in alcoholic liver disease leading to liver cancer. Hepatoma Res 2020;6:5.
6. Romeo I, Marascio N, Pavia G, et al. Structural modeling of new polymorphism clusters of HCV polymerase isolated from direct-acting antiviral naïve patients: focus on dasabuvir and setrobuvir binding affinity. ChemistrySelect 2018;3:6009-17.
7. Marascio N, Pavia G, Romeo I, et al. Real-life 3D therapy failure: analysis of NS5A 93H RAS plus 108 K polymorphism in complex with ombitasvir by molecular modeling. J Med Virol 2018;90:1257-63.
8. Marascio N, Mazzitelli M, Pavia G, et al. Clinical, virological characteristics, and outcomes of treatment with sofosbuvir/ledipasvir in two pediatric patients infected by HCV genotype 4. Cells 2019;8:416.
9. Marascio N, Mazzitelli M, Scarlata GG, et al. HCV antibody prevalence and genotype evolution in a teaching hospital, Calabria region, Southern Italy over a decade (2008-2018). TOMICROJ 2020;14:84-90.
10. Wong GL, Hui VW, Yip TC, et al. Universal HBV vaccination dramatically reduces the prevalence of HBV infection and incidence of hepatocellular carcinoma. Aliment Pharmacol Ther 2022;56:869-77.
11. Masarone M, Federico A, Abenavoli L, Loguercio C, Persico M. Non alcoholic fatty liver: epidemiology and natural history. Rev Recent Clin Trials 2014;9:126-33.
12. Zhang P. Influence of Foods and Nutrition on the Gut Microbiome and Implications for Intestinal Health. Int J Mol Sci 2022;23:9588.
13. Abenavoli L, Scarlata GGM, Paravati MR, Boccuto L, Luzza F, Scarpellini E. Gut microbiota and liver transplantation: immune mechanisms behind the rejection. Biomedicines 2023;11:1792.
14. Gopalakrishnan V, Helmink BA, Spencer CN, Reuben A, Wargo JA. The influence of the gut microbiome on cancer, immunity, and cancer immunotherapy. Cancer Cell 2018;33:570-80.
15. Abenavoli L, Montori M, Svegliati Baroni G, et al. Perspective on the role of gut microbiome in the treatment of hepatocellular carcinoma with immune checkpoint inhibitors. Medicina 2023;59:1427.
16. Maestri M, Santopaolo F, Pompili M, Gasbarrini A, Ponziani FR. Gut microbiota modulation in patients with non-alcoholic fatty liver disease: effects of current treatments and future strategies. Front Nutr 2023;10:1110536.
17. Tripathi A, Debelius J, Brenner DA, et al. The gut-liver axis and the intersection with the microbiome. Nat Rev Gastroenterol Hepatol 2018;15:397-411.
18. Wang Y, Pan CQ, Xing H. Advances in gut microbiota of viral hepatitis cirrhosis. Biomed Res Int 2019;2019:9726786.
19. Abenavoli L, Di Renzo L, Boccuto L, Alwardat N, Gratteri S, De Lorenzo A. Health benefits of Mediterranean diet in nonalcoholic fatty liver disease. Expert Rev Gastroenterol Hepatol 2018;12:873-81.
20. Plaz Torres MC, Aghemo A, Lleo A, et al. Mediterranean diet and NAFLD: what we know and questions that still need to be answered. Nutrients 2019;11:2971.
21. Abenavoli L, Scarpellini E, Pellicano R, Fagoonee S, Larussa T, Luzza F. Mediterranean diet and probiotics supplementation to treat non-alcoholic fatty liver disease. Minerva Med 2020;111:526-8.
22. Abenavoli L, Procopio AC, Paravati MR, et al. Mediterranean diet: the beneficial effects of lycopene in non-alcoholic fatty liver disease. J Clin Med 2022;11:3477.
23. Zohoori F. Chapter 1: Nutrition and diet. In: Zohoori FV, Duckworth RM, editors. The Impact of Nutrition and Diet on Oral Health. S. Karger AG; 2020. pp. 1-13.
24. Rowland I, Gibson G, Heinken A, et al. Gut microbiota functions: metabolism of nutrients and other food components. Eur J Nutr 2018;57:1-24.
25. Ruiz-Margáin A, Román-Calleja BM, Moreno-Guillén P, et al. Nutritional therapy for hepatocellular carcinoma. World J Gastrointest Oncol 2021;13:1440-52.
26. Malmir H, Mahdavi FS, Ejtahed HS, et al. Junk food consumption and psychological distress in children and adolescents: a systematic review and meta-analysis. Nutr Neurosci 2023;26:807-27.
27. Bohara SS, Thapa K, Bhatt LD, Dhami SS, Wagle S. Determinants of junk food consumption among adolescents in Pokhara valley, Nepal. Front Nutr 2021;8:644650.
28. Hymel E, Vlock E, Fisher KW, Farazi PA. Differential progression of unhealthy diet-induced hepatocellular carcinoma in obese and non-obese mice. PLoS One 2022;17:e0272623.
29. Bojková B, Winklewski PJ, Wszedybyl-Winklewska M. Dietary fat and cancer-which is good, which is bad, and the body of evidence. Int J Mol Sci 2020;21:4114.
30. Masood W, Annamaraju P, Khan Suheb MZ, Uppaluri KR. Ketogenic diet. Available from: https://www.ncbi.nlm.nih.gov/books/NBK499830/ [Last accessed on 23 Apr 2024].
31. Luo W, Zhang J, Xu D, et al. Low carbohydrate ketogenic diets reduce cardiovascular risk factor levels in obese or overweight patients with T2DM: a meta-analysis of randomized controlled trials. Front Nutr 2022;9:1092031.
32. Pietrzak D, Kasperek K, Rękawek P, Piątkowska-Chmiel I. The therapeutic role of ketogenic diet in neurological disorders. Nutrients 2022;14:1952.
33. Danan A, Westman EC, Saslow LR, Ede G. The ketogenic diet for refractory mental illness: a retrospective analysis of 31 inpatients. Front Psychiatry 2022;13:951376.
34. Landry MJ, Crimarco A, Perelman D, et al. Adherence to ketogenic and mediterranean study diets in a crossover trial: the keto-med randomized trial. Nutrients 2021;13:967.
35. Healy ME, Chow JD, Byrne FL, et al. Dietary effects on liver tumor burden in mice treated with the hepatocellular carcinogen diethylnitrosamine. J Hepatol 2015;62:599-606.
36. Huang D, Li T, Wang L, et al. Hepatocellular carcinoma redirects to ketolysis for progression under nutrition deprivation stress. Cell Res 2016;26:1112-30.
37. Byrne FL, Hargett SR, Lahiri S, et al. Serial MRI imaging reveals minimal impact of ketogenic diet on established liver tumor growth. Cancers 2018;10:312.
38. Motta J, Lobo L, Fabiola D. Ketogenic diet against liver tumor. Current Developments in Nutrition 2020;4:nzaa044_037.
39. Davis C, Bryan J, Hodgson J, Murphy K. Definition of the mediterranean diet; a literature review. Nutrients 2015;7:9139-53.
40. Merra G, Noce A, Marrone G, et al. Influence of mediterranean diet on human gut microbiota. Nutrients 2020;13:7.
41. Schwingshackl L, Morze J, Hoffmann G. Mediterranean diet and health status: active ingredients and pharmacological mechanisms. Br J Pharmacol 2020;177:1241-57.
42. Abenavoli L, Boccuto L, Federico A, et al. Diet and non-alcoholic fatty liver disease: the mediterranean way. Int J Environ Res Public Health 2019;16:3011.
43. Colica C, DE Lorenzo A, Abenavoli L. Mediterranean diet in liver steatosis: the role of polyphenols. Minerva Gastroenterol Dietol 2018;64:97-9.
44. Calabrò A, Procopio AC, Primerano F, et al. Beneficial effects of coffee in non-alcoholic fatty liver disease: a narrative review. Hepatoma Res 2020;6:69.
45. Turati F, Trichopoulos D, Polesel J, et al. Mediterranean diet and hepatocellular carcinoma. J Hepatol 2014;60:606-11.
46. Li WQ, Park Y, McGlynn KA, et al. Index-based dietary patterns and risk of incident hepatocellular carcinoma and mortality from chronic liver disease in a prospective study. Hepatology 2014;60:588-97.
47. Ma Y, Yang W, Simon TG, et al. Dietary patterns and risk of hepatocellular carcinoma among U.S. men and women. Hepatology 2019;70:577-86.
48. Bogumil D, Park SY, Le Marchand L, et al. High-quality diets are associated with reduced risk of hepatocellular carcinoma and chronic liver disease: the multiethnic cohort. Hepatol Commun 2019;3:437-47.
49. Mentella MC, Scaldaferri F, Ricci C, Gasbarrini A, Miggiano GAD. Cancer and mediterranean diet: a review. Nutrients 2019;11:2059.
50. Procopio AC, Paravati MR, Scarlata GGM, et al. Is the mediterranean diet a good preventive measure for NASH? Hepatoma Res 2023;10:9.
51. Liu K, Verset G, Trepo E, Seth D. Genetics of alcohol-related hepatocellular carcinoma - its role in risk prediction. Hepatoma Res 2020;6:42.
52. Teschke R. Hepatocellular carcinoma in alcoholic liver disease: mechanistic considerations and clinical facts. Hepatoma Res 2019;5:40.
53. Chen P, Li Y, Dai Y, et al. Advances in the pathogenesis of metabolic liver disease-related hepatocellular carcinoma. J Hepatocell Carcinoma 2024;11:581-94.
54. Puoti C. New insights on hepatocellular carcinoma: epidemiology and clinical aspects. Hepatoma Res 2018;4:57.
55. Geh D, Anstee QM, Reeves HL. NAFLD-associated HCC: progress and opportunities. J Hepatocell Carcinoma 2021;8:223-39.
56. Hou K, Wu ZX, Chen XY, et al. Microbiota in health and diseases. Signal Transduct Target Ther 2022;7:135.
57. Ghosh SS, Wang J, Yannie PJ, Ghosh S. Intestinal barrier dysfunction, LPS translocation, and disease development. J Endocr Soc 2020;4:bvz039.
58. Temraz S, Nassar F, Kreidieh F, Mukherji D, Shamseddine A, Nasr R. Hepatocellular carcinoma immunotherapy and the potential influence of gut microbiome. Int J Mol Sci 2021;22:7800.
59. Abenavoli L, Scarlata GGM, Scarpellini E, et al. Metabolic-dysfunction-associated fatty liver disease and gut microbiota: from fatty liver to dysmetabolic syndrome. Medicina 2023;59:594.
60. Marascio N, De Caro C, Quirino A, et al. The role of the microbiota gut-liver axis during HCV chronic infection: a schematic overview. J Clin Med 2022;11:5936.
61. Ponziani FR, Bhoori S, Castelli C, et al. Hepatocellular carcinoma is associated with gut microbiota profile and inflammation in nonalcoholic fatty liver disease. Hepatology 2019;69:107-20.
62. Effenberger M, Waschina S, Bronowski C, et al. A gut bacterial signature in blood and liver tissue characterizes cirrhosis and hepatocellular carcinoma. Hepatol Commun 2023;7:e00182.
63. Ma J, Li J, Jin C, et al. Association of gut microbiome and primary liver cancer: a two-sample mendelian randomization and case-control study. Liver Int 2023;43:221-33.
64. Yang S, Dai H, Lu Y, Li R, Gao C, Pan S. Trimethylamine N-oxide promotes cell proliferation and angiogenesis in colorectal cancer. J Immunol Res 2022;2022:7043856.
65. Vétizou M, Pitt JM, Daillère R, et al. Anticancer immunotherapy by CTLA-4 blockade relies on the gut microbiota. Science 2015;350:1079-84.
66. Ayers M, Lunceford J, Nebozhyn M, et al. IFN-γ-related mRNA profile predicts clinical response to PD-1 blockade. J Clin Invest 2017;127:2930-40.
67. Tanoue T, Morita S, Plichta DR, et al. A defined commensal consortium elicits CD8 T cells and anti-cancer immunity. Nature 2019;565:600-5.
68. Zheng Y, Wang T, Tu X, et al. Gut microbiome affects the response to anti-PD-1 immunotherapy in patients with hepatocellular carcinoma. J Immunother Cancer 2019;7:193.
69. Lee PC, Wu CJ, Hung YW, et al. Gut microbiota and metabolites associate with outcomes of immune checkpoint inhibitor-treated unresectable hepatocellular carcinoma. J Immunother Cancer 2022;10:e004779.
70. Yoshimoto S, Loo TM, Atarashi K, et al. Obesity-induced gut microbial metabolite promotes liver cancer through senescence secretome. Nature 2013;499:97-101.
71. Luo W, Guo S, Zhou Y, et al. Hepatocellular carcinoma: how the gut microbiota contributes to pathogenesis, diagnosis, and therapy. Front Microbiol 2022;13:873160.
72. Ganesan R, Yoon SJ, Suk KT. Microbiome and metabolomics in liver cancer: scientific technology. Int J Mol Sci 2022;24:537.
73. Wan MLY, El-Nezami H. Targeting gut microbiota in hepatocellular carcinoma: probiotics as a novel therapy. Hepatobiliary Surg Nutr 2018;7:11-20.
74. Ma C, Han M, Heinrich B, et al. Gut microbiome-mediated bile acid metabolism regulates liver cancer via NKT cells. Science 2018;360:eaan5931.
75. Yamada S, Takashina Y, Watanabe M, et al. Bile acid metabolism regulated by the gut microbiota promotes non-alcoholic steatohepatitis-associated hepatocellular carcinoma in mice. Oncotarget 2018;9:9925-39.
76. Van Rooyen DM, Larter CZ, Haigh WG, et al. Hepatic free cholesterol accumulates in obese, diabetic mice and causes nonalcoholic steatohepatitis. Gastroenterology 2011;141:1393-403, 1403.e1.
77. Liu D, Wong CC, Fu L, et al. Squalene epoxidase drives NAFLD-induced hepatocellular carcinoma and is a pharmaceutical target. Sci Transl Med 2018;10:eaap9840.
78. McGettigan B, McMahan R, Orlicky D, et al. Dietary lipids differentially shape nonalcoholic steatohepatitis progression and the transcriptome of kupffer cells and infiltrating macrophages. Hepatology 2019;70:67-83.
79. Zhang X, Coker OO, Chu ES, et al. Dietary cholesterol drives fatty liver-associated liver cancer by modulating gut microbiota and metabolites. Gut 2021;70:761-74.
80. Zhou F, Sun X. Cholesterol metabolism: a double-edged sword in hepatocellular carcinoma. Front Cell Dev Biol 2021;9:762828.
81. Zhao S, Jang C, Liu J, et al. Dietary fructose feeds hepatic lipogenesis via microbiota-derived acetate. Nature 2020;579:586-91.
82. Pan Y, Zhang X. Diet and gut microbiome in fatty liver and its associated liver cancer. J Gastroenterol Hepatol 2022;37:7-14.
83. Moussa I, Day RS, Li R, et al. Association of dietary fat intake and hepatocellular carcinoma among US adults. Cancer Med 2021;10:7308-19.
84. Chang Y, Liu Q, Zhou Z, et al. Can statin treatment reduce the risk of hepatocellular carcinoma? Technol Cancer Res Treat 2020;19:1533033820934881.
85. Russo A, Serapide F, Quirino A, et al. Microbiological and clinical findings of SARS-CoV-2 infection after 2 years of pandemic: from lung to gut microbiota. Diagnostics 2022;12:2143.
86. Abenavoli L, Scarpellini E, Paravati MR, et al. Gut microbiota and critically ill patients: immunity and its modulation via probiotics and immunonutrition. Nutrients 2023;15:3569.
87. Marascio N, Scarlata GGM, Romeo F, et al. The role of gut microbiota in the clinical outcome of septic patients: state of the art and future perspectives. Int J Mol Sci 2023;24:9307.
88. Claesson MJ, Clooney AG, O'Toole PW. A clinician’s guide to microbiome analysis. Nat Rev Gastroenterol Hepatol 2017;14:585-95.
89. Odrzywolek K, Karwowska Z, Majta J, Byrski A, Milanowska-Zabel K, Kosciolek T. Deep embeddings to comprehend and visualize microbiome protein space. Sci Rep 2022;12:10332.
90. Cammarota G, Ianiro G, Ahern A, et al. Gut microbiome, big data and machine learning to promote precision medicine for cancer. Nat Rev Gastroenterol Hepatol 2020;17:635-48.
91. Sundh J, Tanash H, Arian R, et al. Advanced dental cleaning is associated with reduced risk of COPD exacerbations - a randomized controlled trial. Int J Chron Obstruct Pulmon Dis 2021;16:3203-15.
92. Wirbel J, Zych K, Essex M, et al. Microbiome meta-analysis and cross-disease comparison enabled by the SIAMCAT machine learning toolbox. Genome Biol 2021;22:93.
Cite This Article
Export citation file: BibTeX | RIS
OAE Style
Scarlata GGM, Cicino C, Spagnuolo R, Marascio N, Quirino A, Matera G, Dumitrașcu DL, Luzza F, Abenavoli L. Impact of diet and gut microbiota changes in the development of hepatocellular carcinoma. Hepatoma Res 2024;10:19. http://dx.doi.org/10.20517/2394-5079.2023.122
AMA Style
Scarlata GGM, Cicino C, Spagnuolo R, Marascio N, Quirino A, Matera G, Dumitrașcu DL, Luzza F, Abenavoli L. Impact of diet and gut microbiota changes in the development of hepatocellular carcinoma. Hepatoma Research. 2024; 10: 19. http://dx.doi.org/10.20517/2394-5079.2023.122
Chicago/Turabian Style
Scarlata, Giuseppe Guido Maria, Claudia Cicino, Rocco Spagnuolo, Nadia Marascio, Angela Quirino, Giovanni Matera, Dan Lucian Dumitrașcu, Francesco Luzza, Ludovico Abenavoli. 2024. "Impact of diet and gut microbiota changes in the development of hepatocellular carcinoma" Hepatoma Research. 10: 19. http://dx.doi.org/10.20517/2394-5079.2023.122
ACS Style
Scarlata, GGM.; Cicino C.; Spagnuolo R.; Marascio N.; Quirino A.; Matera G.; Dumitrașcu DL.; Luzza F.; Abenavoli L. Impact of diet and gut microbiota changes in the development of hepatocellular carcinoma. Hepatoma. Res. 2024, 10, 19. http://dx.doi.org/10.20517/2394-5079.2023.122
About This Article
Special Issue
Copyright
Data & Comments
Data
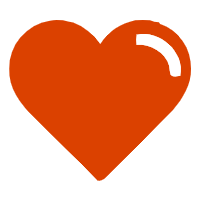

Comments
Comments must be written in English. Spam, offensive content, impersonation, and private information will not be permitted. If any comment is reported and identified as inappropriate content by OAE staff, the comment will be removed without notice. If you have any queries or need any help, please contact us at support@oaepublish.com.